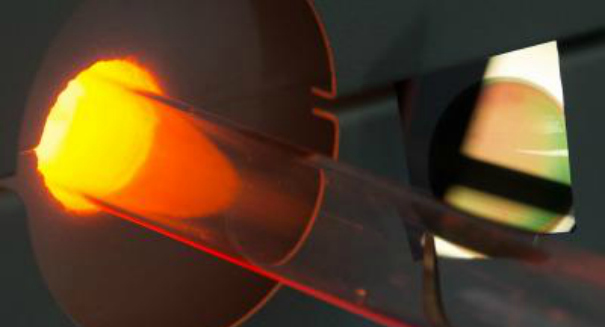
Instead of keeping energy in chemical reactions the way batteries do, supercapacitors keep electricity by gathering ions on the surface of a porous material.
According to a news release from Vanderbilt University, a new device created by scientists at the university stores electricity on silicon chips. The new supercapacitor design may lead to solar cells that generate electricity 24/7 and cell phones with built-in power cells that recharge in seconds and last for weeks.
According to scientists, it is the first supercapacitor constructed out of silicon so it can be placed into a silicon chip along with the microelectronic circuitry that it powers. They add that it is likely that these power cells can be made out of the leftover silicon that exists in the current generation of solar cells, as well as numerous other electromechanical devices, offering a significant cost savings.
“If you ask experts about making a supercapacitor out of silicon, they will tell you it is a crazy idea,” noted Cary Pint, an assistant professor of mechanical engineering at Vanderbilt University, in a statement. “But we’ve found an easy way to do it.”
Instead of keeping energy in chemical reactions the way batteries do, supercapacitors keep electricity by gathering ions on the surface of a porous material. Thus, they tend to charge and discharge in minutes and operate for a few million cycles.
These features have allowed commercial supercapacitors to find success via the storage of energy captured by regenerative braking systems on buses and electric vehicles. However, supercapacitors lack the electrical energy storage capability of lithium-ion batteries.
Efforts to enhance the energy density of supercapacitors have concentrated on carbon-based nanomaterials like graphene and nanotubes. Because these devices keep electrical charge on the surface of their electrodes, the way to raise their energy density is to augment the electrodes’ surface area, which means building surfaces filled with nanoscale ridges and pores.
“The big challenge for this approach is assembling the materials,” Pint posited. “Constructing high-performance, functional devices out of nanoscale building blocks with any level of control has proven to be quite challenging, and when it is achieved it is difficult to repeat.”
The researchers settled on a completely different technique: utilizing porous silicon, a material with a manageable and well-defined nanostructure constructed by electrochemically engraving the surface of a silicon wafer.
This allowed the researchers to make surfaces with optimal nanostructures for supercapacitor electrodes, but it left them with a significant problem. Silicon is usually considered inappropriate for use in supercapacitors because it reacts readily with some of the chemicals in the electrolytes that provide the ions that keep the electrical charge.
The researchers settled on coating the porous silicon surface with carbon.
“We had no idea what would happen,” Pint said. “Typically, researchers grow graphene from silicon-carbide materials at temperatures in excess of 1400 degrees Celsius. But at lower temperatures – 600 to 700 degrees Celsius – we certainly didn’t expect graphene-like material growth.”
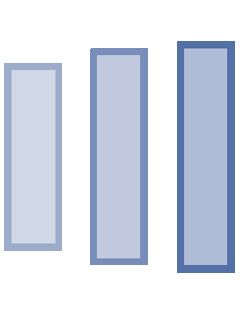
When the researchers took the porous silicon out of the furnace, they discovered that it had transitioned from orange to purple or black. Whey they examined it under a powerful scanning electron microscope they discovered that it appeared nearly identical to the first material but it was coated by a layer of graphene a few nanometers thick.
When the scientist tested the coated material they discovered that it had chemically stabilized the silicon surface. When they utilized it to construct supercapacitors, they discovered that the graphene coating enhanced energy densities by more than two orders of magnitude compared to those constructed from uncoated porous silicon and considerably better than commercial supercapacitors.
The graphene layer behaves like an atomically thin protective coating. The researchers believe that this technique isn’t limited to graphene.
“The ability to engineer surfaces with atomically thin layers of materials combined with the control achieved in designing porous materials opens opportunities for a number of different applications beyond energy storage,” Pint argued.
“Despite the excellent device performance we achieved, our goal wasn’t to create devices with record performance,” he added. “It was to develop a road map for integrated energy storage. Silicon is an ideal material to focus on because it is the basis of so much of our modern technology and applications. In addition, most of the silicon in existing devices remains unused since it is very expensive and wasteful to produce thin silicon wafers.”
The researchers are currently utilizing this method to create energy storage that can be developed in the excess materials or on the unused back sides of solar cells and sensors. The supercapacitors would keep excess electrically that the cells produce at midday and release it when the demand spikes in the afternoon.
“All the things that define us in a modern environment require electricity,” Pint concluded. “The more that we can integrate power storage into existing materials and devices, the more compact and efficient they will become.”
The study’s findings are described in greater detail in the journal Scientific Reports.
Leave a Reply